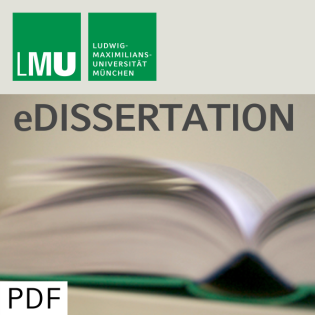
The Production and Investigation of Cold Antihydrogen Atoms
Beschreibung
vor 19 Jahren
This work reports on experiments in which antihydrogen atoms have
been produced in cryogenic Penning traps from antiproton and
positron plasmas by two different methods and on experiments that
have been carried out subsequently in order to investigate the
antihydrogen atoms. By the first method antihydrogen atoms have
been formed during the process of positron cooling of antiprotons
in so called nested Penning traps and detected via a field
ionization method. A linear dependence of the number of detected
antihydrogen atoms on the number of positrons has been found. A
measurement of the state distribution has revealed that the
antihydrogen atoms are formed in highly excited states. This
suggests along with the high production rate that the antihydrogen
atoms are formed by three-body recombination processes and
subsequent collisional deexcitations. However current theory cannot
yet account for the measured state distribution. Typical radii of
the detected antihydrogen atoms lie in the range between 0.4 µm and
0.15 µm. The deepest bound antihydrogen atoms have radii below 0.1
µm. Antihydrogen atoms with that size have chaotic positron orbits
so that for the first time antihydrogen atoms have been detected
that cannot be described by the GCA-model. The kinetic energy of
the weakest bound antihydrogen atoms has been measured to about 200
meV, which corresponds to an antihydrogen velocity of approximately
6200 m/s. A simple model suggests that these atoms are formed from
only one deexcitation collision and methods that might lead to a
decrease of the antihydrogen velocity are presented. By the second
method antihydrogen atoms have been synthesized in charge-exchange
processes. Lasers are used to produce a Rydberg cesium beam within
the cryogenic Penning trap that collides with trapped positrons so
that Rydberg positronium atoms are formed via charge-exchange
reactions. Due to their charge neutrality the Rydberg positronium
atoms are free to leave the positron trapping region. The Rydberg
positronium atoms that collide with nearby stored antiprotons form
antihydrogen atoms in charge-exchange reactions. So far, 14 +/- 4
antihydrogen atoms have been detected background-free via a
field-ionization method. The antihydrogen atoms produced via the
two-step charge-exchange mechanism are expected to have a
temperature of 4.2 K, the temperature of the antiprotons from which
they are formed. A method is proposed by which the antihydrogen
temperature can be determined with an accuracy of better than 1 K
from a measurement of the time delay between antihydrogen
annihilation events and the laser pulse that initiates the
antihydrogen production via the production of Rydberg cesium atoms.
First experiments have been carried out during the last days of the
2004 beam time, but the number of detected antihydrogen
annihilations has been too low for a determination of the
antihydrogen temperature. Trapped antiprotons have been directly
exposed to laser light delivered by a Titanium:Sapphire laser in
order to investigate if the laser light causes any loss on the
trapped antiprotons. Experiments have shown that no extra loss
occurs for laser powers of less than 590 mW. This is an important
result against the background of the future plan to confine
antihydrogen atoms in a combined Penning-Ioffe trap and then to
carry out laser spectroscopy on these atoms, since it reveals that
laser light does not cause an increase of the pressure in the
trapping region to the extend that annihilations with the
background gas become noticeable. The ATRAP Collaboration plans to
precisely investigate antihydrogen atoms. The ultimate goal is to
test the CPT-theorem by a high precision measurement of the 1S-2S
transition of antihydrogen and a comparison with the precisely
known value of the corresponding transition in hydrogen. This
thesis presents the achievement of the first step towards this
challenging goal: the production of cold antihydrogen itself.
been produced in cryogenic Penning traps from antiproton and
positron plasmas by two different methods and on experiments that
have been carried out subsequently in order to investigate the
antihydrogen atoms. By the first method antihydrogen atoms have
been formed during the process of positron cooling of antiprotons
in so called nested Penning traps and detected via a field
ionization method. A linear dependence of the number of detected
antihydrogen atoms on the number of positrons has been found. A
measurement of the state distribution has revealed that the
antihydrogen atoms are formed in highly excited states. This
suggests along with the high production rate that the antihydrogen
atoms are formed by three-body recombination processes and
subsequent collisional deexcitations. However current theory cannot
yet account for the measured state distribution. Typical radii of
the detected antihydrogen atoms lie in the range between 0.4 µm and
0.15 µm. The deepest bound antihydrogen atoms have radii below 0.1
µm. Antihydrogen atoms with that size have chaotic positron orbits
so that for the first time antihydrogen atoms have been detected
that cannot be described by the GCA-model. The kinetic energy of
the weakest bound antihydrogen atoms has been measured to about 200
meV, which corresponds to an antihydrogen velocity of approximately
6200 m/s. A simple model suggests that these atoms are formed from
only one deexcitation collision and methods that might lead to a
decrease of the antihydrogen velocity are presented. By the second
method antihydrogen atoms have been synthesized in charge-exchange
processes. Lasers are used to produce a Rydberg cesium beam within
the cryogenic Penning trap that collides with trapped positrons so
that Rydberg positronium atoms are formed via charge-exchange
reactions. Due to their charge neutrality the Rydberg positronium
atoms are free to leave the positron trapping region. The Rydberg
positronium atoms that collide with nearby stored antiprotons form
antihydrogen atoms in charge-exchange reactions. So far, 14 +/- 4
antihydrogen atoms have been detected background-free via a
field-ionization method. The antihydrogen atoms produced via the
two-step charge-exchange mechanism are expected to have a
temperature of 4.2 K, the temperature of the antiprotons from which
they are formed. A method is proposed by which the antihydrogen
temperature can be determined with an accuracy of better than 1 K
from a measurement of the time delay between antihydrogen
annihilation events and the laser pulse that initiates the
antihydrogen production via the production of Rydberg cesium atoms.
First experiments have been carried out during the last days of the
2004 beam time, but the number of detected antihydrogen
annihilations has been too low for a determination of the
antihydrogen temperature. Trapped antiprotons have been directly
exposed to laser light delivered by a Titanium:Sapphire laser in
order to investigate if the laser light causes any loss on the
trapped antiprotons. Experiments have shown that no extra loss
occurs for laser powers of less than 590 mW. This is an important
result against the background of the future plan to confine
antihydrogen atoms in a combined Penning-Ioffe trap and then to
carry out laser spectroscopy on these atoms, since it reveals that
laser light does not cause an increase of the pressure in the
trapping region to the extend that annihilations with the
background gas become noticeable. The ATRAP Collaboration plans to
precisely investigate antihydrogen atoms. The ultimate goal is to
test the CPT-theorem by a high precision measurement of the 1S-2S
transition of antihydrogen and a comparison with the precisely
known value of the corresponding transition in hydrogen. This
thesis presents the achievement of the first step towards this
challenging goal: the production of cold antihydrogen itself.
Weitere Episoden

vor 16 Jahren
In Podcasts werben
Kommentare (0)