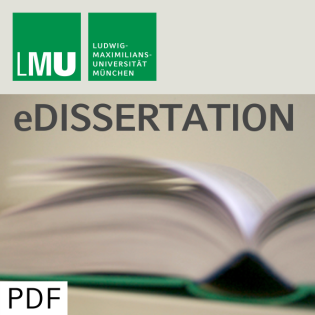
Solar radiative transfer parameterizations for three-dimensional effects in cloudy atmospheres
Beschreibung
vor 17 Jahren
This thesis addresses two major problems in the field of radiative
transfer (RT) in the earth’s atmosphere. The first problem is
linked with the need for significant computational resources of RT
in a three-dimensional (3D) atmospheric model. Although only highly
efficient one-dimensional (1D) RT models are employed for each
pixel of the model domain separately and independently, it is still
not possible to utilize these models on a frequent basis, compared
to the rate at which meteorological variables are computed. That
means that the calculated radiative properties (RP) are held
constant for a longer period of time, while the prognostic
meteorological variables are updated at a rapid rate. Even though
there is no detailed study about the consequences of this
disproportion, an attempt was made to develop an RT model which
permits the fast computation of basic radiative transfer properties
which could be used in the future to update this information more
frequently. The developed model is based on the application of the
radiative transfer perturbation theory to realistic cloud fields
column by column. It turned out that the application, intended to
replace the Independent Pixel Approximation (IPA), see below, is
possible and promising within the assumptions and constraints of
the utilized methods. It could be demonstrated that, depending on
the actual case, errors in the pixel transmission and reflection
stay bounded to values of up to 10%−15%. In one case the achieved
acceleration could be investigated. It was about a factor of four
compared to the direct application of the usual forward variant of
the model, although no numerical optimization was carried out. The
second problem concerns the realistic treatment of the 3D
interactions of clouds and solar radiation. As implied in the above
paragraph, 1D RT models are usually employed column by column which
suppresses the exchange of radiation between those columns. Thus,
fundamental 3D effects are neglected by this so-called Independent
Pixel Approximation (IPA). These comprise not only small scale
contributions due to diffuse radiative transport, but also large
scale patterns like geometric effects of the inclined solar
illumination. Examples are blurred radiative structures due to
radiative smoothing and the shifted location of shadows and bright
areas. To parameterize those effects strong efforts have been
undertaken during the last couple of years. However, no method has
proven to be completely satisfactory and ready for implementation.
To carry this research one step further two approaches have been
adopted and extended. The first is the concept of the Tilted
Independent Pixel Approximation (TIPA). In contrast to the IPA,
which ignores the solar geometry, this method correctly accounts
for the slant illumination due to the correct tracking of the
direct beam. As a result, the optical parameters in the slant
columns are arranged in a more realistic order and the attenuation
and the positions of the RP are less erroneous. To further improve
this method a transformation has been developed which yields 3D
resolution of the RP in the original grid. Since the TIPA still
does not include any diffuse radiative exchange as another approach
the Nonlocal Independent Pixel Approximation (NIPA) has been
explored. This technique uses 1D results and carries out a
convolution product to distribute RP across column boundaries. In
order to arrive at a fully independent treatment of this method a
simplified derivation of the convolution parameters was developed.
Finally, TIPA and NIPA are combined to form NTIPA. These approaches
have proven to be superior to IPA with respect to several aspects.
The improvement ranges from several percent to 50% if maximum
errors of the transmitted and reflected light are considered.
Criteria like the distribution of the errors or the vertical
profiles of the RP are also more preferable than their counterparts
derived by IPA.
transfer (RT) in the earth’s atmosphere. The first problem is
linked with the need for significant computational resources of RT
in a three-dimensional (3D) atmospheric model. Although only highly
efficient one-dimensional (1D) RT models are employed for each
pixel of the model domain separately and independently, it is still
not possible to utilize these models on a frequent basis, compared
to the rate at which meteorological variables are computed. That
means that the calculated radiative properties (RP) are held
constant for a longer period of time, while the prognostic
meteorological variables are updated at a rapid rate. Even though
there is no detailed study about the consequences of this
disproportion, an attempt was made to develop an RT model which
permits the fast computation of basic radiative transfer properties
which could be used in the future to update this information more
frequently. The developed model is based on the application of the
radiative transfer perturbation theory to realistic cloud fields
column by column. It turned out that the application, intended to
replace the Independent Pixel Approximation (IPA), see below, is
possible and promising within the assumptions and constraints of
the utilized methods. It could be demonstrated that, depending on
the actual case, errors in the pixel transmission and reflection
stay bounded to values of up to 10%−15%. In one case the achieved
acceleration could be investigated. It was about a factor of four
compared to the direct application of the usual forward variant of
the model, although no numerical optimization was carried out. The
second problem concerns the realistic treatment of the 3D
interactions of clouds and solar radiation. As implied in the above
paragraph, 1D RT models are usually employed column by column which
suppresses the exchange of radiation between those columns. Thus,
fundamental 3D effects are neglected by this so-called Independent
Pixel Approximation (IPA). These comprise not only small scale
contributions due to diffuse radiative transport, but also large
scale patterns like geometric effects of the inclined solar
illumination. Examples are blurred radiative structures due to
radiative smoothing and the shifted location of shadows and bright
areas. To parameterize those effects strong efforts have been
undertaken during the last couple of years. However, no method has
proven to be completely satisfactory and ready for implementation.
To carry this research one step further two approaches have been
adopted and extended. The first is the concept of the Tilted
Independent Pixel Approximation (TIPA). In contrast to the IPA,
which ignores the solar geometry, this method correctly accounts
for the slant illumination due to the correct tracking of the
direct beam. As a result, the optical parameters in the slant
columns are arranged in a more realistic order and the attenuation
and the positions of the RP are less erroneous. To further improve
this method a transformation has been developed which yields 3D
resolution of the RP in the original grid. Since the TIPA still
does not include any diffuse radiative exchange as another approach
the Nonlocal Independent Pixel Approximation (NIPA) has been
explored. This technique uses 1D results and carries out a
convolution product to distribute RP across column boundaries. In
order to arrive at a fully independent treatment of this method a
simplified derivation of the convolution parameters was developed.
Finally, TIPA and NIPA are combined to form NTIPA. These approaches
have proven to be superior to IPA with respect to several aspects.
The improvement ranges from several percent to 50% if maximum
errors of the transmitted and reflected light are considered.
Criteria like the distribution of the errors or the vertical
profiles of the RP are also more preferable than their counterparts
derived by IPA.
Weitere Episoden

vor 16 Jahren
In Podcasts werben
Kommentare (0)