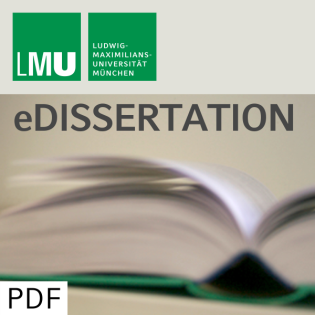
Human checkpoint proteins hRad9, hHus1, and hRad1 form a DNA damage-responsive complex
Beschreibung
vor 20 Jahren
Human cells have evolved protective mechanisms such as DNA repair
and cell cycle checkpoints in order to promote stability of the
genome. Studies on hereditary instability syndromes associated with
a higher incidence of malignancies like Xeroderma pigmentosum or
Nijmegen breakage syndrome demonstrated that genetic defects and
subsequent dysfunction of a specific DNA repair mechanism trigger
the development of cancer. Within the last years, the investigation
of cell cycle checkpoints gained increasing importance in cancer
research. Checkpoints are signaling cascades that halt the cell
cycle in response to DNA damage, thereby providing time for repair
and preventing accumulation of DNA alterations. While the
p53-dependent G1-S checkpoint has been extensively investigated,
little is known about other checkpoints in humans such as the G2-M
or the S-phase progression checkpoint. Studies on the human cancer
syndrome ataxia telangiectasia (AT) showed that AT cells fail to
induce several checkpoints in response to ionizing radiation (IR),
indicating that a checkpoint gene defect is responsible for the
AT-associated cancers. The responsible gene (ATM) has significant
sequence homology to the checkpoint kinase gene sprad3 in the
fission yeast Schizosaccharomyces pombe (S. pombe). In S. pombe,
spRad3 regulates G2-M checkpoint activation in response to DNA
damage. Defects in the sprad3 gene, like defects in ATM, sensitize
the organisms to radiation and radiomimetic drugs, suggesting
conservation of checkpoint pathways from yeast to humans as well as
a potential role of the G2-M checkpoint in carcinogenesis. The
discovery of G2-M checkpoint-deficient yeast mutants led to the
cloning of additional checkpoint genes in yeast and their human
homologs. This group of novel human genes includes homologs of
sprad9 (hRAD9), sphus1 (hHUS1), and sprad1 (hRAD1). In S. pombe,
these genes are required for activation of spRad3, and defects in
one or more of these genes render the yeast more sensitive to
genotoxic agents. Mutations within the human rad genes may bring
about an increased rate of mutations and genomic instability as
shown for p53 or AT and may be responsible for inherited
predisposition to cancer. In view of this potential importance of
human rad genes in the process of carcinogenesis, we have
undertaken a cellular and molecular analysis of the novel human
checkpoint proteins hRad9, hHus1, and hRad1 in the leukemia cell
line K562 and in human keratinocytes. Using specific antibodies to
the hRad9, hHus1, and hRad1 proteins we demonstrated with
co-immunoprecipitation and Western-blot experiments that the human
checkpoint proteins hRad9, hHus1, and hRad1 associate in a
biochemical complex similar to the spRad9-spHus1-spRad1 complex
reported in fission yeast. To generate a model system of checkpoint
protein function amenable to biochemical analysis, we prepared
epitope-tagged expression vectors for hRad9, hHus1, and hRad1,
which were transfected into K562 cells by electroporation,
resulting in transient expression of epitope-tagged protein. By
simultaneous expression of hRad9, hHus1, and hRad1 we showed that
transiently expressed epitope-tagged checkpoint proteins hRad9,
hHus1, and hRad1 recapitulate complex formation of endogenous
proteins. Immunoprecipitation studies with lysates of
hRad9-overexpressing cells revealed that hRad9 undergoes complex
post-translational modifications. Co-expression of hRad9 with
hHus1, and hRad1 resulted in a large increase of the amount of a
highly modified form of hRad9, suggesting that hRad1 and hHus1
either promote formation of, or stabilize the modified form of
hRad9. Previously, a direct correlation between checkpoint protein
phosphorylation and activation of DNA damage checkpoints in yeast
was proposed. In this study, we show that hRad9 is phosphorylated
in response to DNA damage, and that phosphorylated hRad9 interacts
with hHus1 and hRad1 as well. The present results suggest that the
hRad9-hHus1-hRad1 complex actively participates in an
evolutionarily conserved DNA damage-induced signaling cascade.
hRad1 seems to possess exonuclease activity. The presence of a
putative DNA-metabolizing protein in the multimolecular checkpoint
complex, coupled with genetic data that place spRad9, spHus1, and
spRad1 early in the response pathway of checkpoint activation
suggests that the complex may function as a sensor that scans the
genome for damaged DNA. Once damaged DNA is detected, this complex
may initiate endonucleolytic processing of the lesions and trigger
interactions with downstream signaling elements, or may link
unknown damage recognition components to downstream
signal-transducing pathways that include the ATM kinase, which is
implicated in actively enforcing cell cycle arrest after DNA
damage. Potential goals of checkpoint research include the
implementation of screening tests to identify familial cancer
predisposition and treatment of checkpoint gene defects by gene
transfer. Another aim of checkpoint research is the development of
checkpoint-based cancer therapy. More than 50% of all human
malignant tumors contain mutated p53, and p53-deficient tumor cells
lack induction of the G1-S checkpoint in response to DNA damage.
One emerging hypothesis is that selective inhibitors of the
compensating G2-M checkpoint would preferentially radiosensitize
p53-deficient tumor cells. Thus, the investigation of checkpoint
function in humans provides further targets for chemotherapeutic
agents and will help to design future strategies in cancer therapy.
and cell cycle checkpoints in order to promote stability of the
genome. Studies on hereditary instability syndromes associated with
a higher incidence of malignancies like Xeroderma pigmentosum or
Nijmegen breakage syndrome demonstrated that genetic defects and
subsequent dysfunction of a specific DNA repair mechanism trigger
the development of cancer. Within the last years, the investigation
of cell cycle checkpoints gained increasing importance in cancer
research. Checkpoints are signaling cascades that halt the cell
cycle in response to DNA damage, thereby providing time for repair
and preventing accumulation of DNA alterations. While the
p53-dependent G1-S checkpoint has been extensively investigated,
little is known about other checkpoints in humans such as the G2-M
or the S-phase progression checkpoint. Studies on the human cancer
syndrome ataxia telangiectasia (AT) showed that AT cells fail to
induce several checkpoints in response to ionizing radiation (IR),
indicating that a checkpoint gene defect is responsible for the
AT-associated cancers. The responsible gene (ATM) has significant
sequence homology to the checkpoint kinase gene sprad3 in the
fission yeast Schizosaccharomyces pombe (S. pombe). In S. pombe,
spRad3 regulates G2-M checkpoint activation in response to DNA
damage. Defects in the sprad3 gene, like defects in ATM, sensitize
the organisms to radiation and radiomimetic drugs, suggesting
conservation of checkpoint pathways from yeast to humans as well as
a potential role of the G2-M checkpoint in carcinogenesis. The
discovery of G2-M checkpoint-deficient yeast mutants led to the
cloning of additional checkpoint genes in yeast and their human
homologs. This group of novel human genes includes homologs of
sprad9 (hRAD9), sphus1 (hHUS1), and sprad1 (hRAD1). In S. pombe,
these genes are required for activation of spRad3, and defects in
one or more of these genes render the yeast more sensitive to
genotoxic agents. Mutations within the human rad genes may bring
about an increased rate of mutations and genomic instability as
shown for p53 or AT and may be responsible for inherited
predisposition to cancer. In view of this potential importance of
human rad genes in the process of carcinogenesis, we have
undertaken a cellular and molecular analysis of the novel human
checkpoint proteins hRad9, hHus1, and hRad1 in the leukemia cell
line K562 and in human keratinocytes. Using specific antibodies to
the hRad9, hHus1, and hRad1 proteins we demonstrated with
co-immunoprecipitation and Western-blot experiments that the human
checkpoint proteins hRad9, hHus1, and hRad1 associate in a
biochemical complex similar to the spRad9-spHus1-spRad1 complex
reported in fission yeast. To generate a model system of checkpoint
protein function amenable to biochemical analysis, we prepared
epitope-tagged expression vectors for hRad9, hHus1, and hRad1,
which were transfected into K562 cells by electroporation,
resulting in transient expression of epitope-tagged protein. By
simultaneous expression of hRad9, hHus1, and hRad1 we showed that
transiently expressed epitope-tagged checkpoint proteins hRad9,
hHus1, and hRad1 recapitulate complex formation of endogenous
proteins. Immunoprecipitation studies with lysates of
hRad9-overexpressing cells revealed that hRad9 undergoes complex
post-translational modifications. Co-expression of hRad9 with
hHus1, and hRad1 resulted in a large increase of the amount of a
highly modified form of hRad9, suggesting that hRad1 and hHus1
either promote formation of, or stabilize the modified form of
hRad9. Previously, a direct correlation between checkpoint protein
phosphorylation and activation of DNA damage checkpoints in yeast
was proposed. In this study, we show that hRad9 is phosphorylated
in response to DNA damage, and that phosphorylated hRad9 interacts
with hHus1 and hRad1 as well. The present results suggest that the
hRad9-hHus1-hRad1 complex actively participates in an
evolutionarily conserved DNA damage-induced signaling cascade.
hRad1 seems to possess exonuclease activity. The presence of a
putative DNA-metabolizing protein in the multimolecular checkpoint
complex, coupled with genetic data that place spRad9, spHus1, and
spRad1 early in the response pathway of checkpoint activation
suggests that the complex may function as a sensor that scans the
genome for damaged DNA. Once damaged DNA is detected, this complex
may initiate endonucleolytic processing of the lesions and trigger
interactions with downstream signaling elements, or may link
unknown damage recognition components to downstream
signal-transducing pathways that include the ATM kinase, which is
implicated in actively enforcing cell cycle arrest after DNA
damage. Potential goals of checkpoint research include the
implementation of screening tests to identify familial cancer
predisposition and treatment of checkpoint gene defects by gene
transfer. Another aim of checkpoint research is the development of
checkpoint-based cancer therapy. More than 50% of all human
malignant tumors contain mutated p53, and p53-deficient tumor cells
lack induction of the G1-S checkpoint in response to DNA damage.
One emerging hypothesis is that selective inhibitors of the
compensating G2-M checkpoint would preferentially radiosensitize
p53-deficient tumor cells. Thus, the investigation of checkpoint
function in humans provides further targets for chemotherapeutic
agents and will help to design future strategies in cancer therapy.
Weitere Episoden

In Podcasts werben
Kommentare (0)