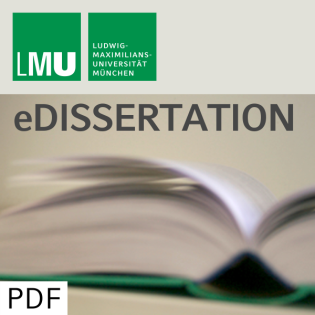
Crystallization of carbonate and sulfate minerals in organic matrices
Beschreibung
vor 9 Jahren
Biological carbonate hard tissues, such as the shell of the bivalve
Mytilus edulis, are composites of biopolymers and minerals. M.
edulis has two distinct layers, the outer layer consists of fibrous
calcite and the inner layer is composed of nacreous aragonite.
Close to the interface between nacreous aragonite and fibrous
calcite, a 1-2 micrometer wide zone exists that consists of
granular aragonite. Aragonite granules and tablets as well as
calcite fibrous are embedded into matrix biopolymers. In order to
understand the composite nature of these hard tissues, biomimetic
experiments using hydrogels were carried out. Hydrogels are able to
model biogenic matrix environments due to their ability to confine
space and to determine diffusion rates, local concentrations and
supersaturation of the solutes. Hydrogels have local
crystallization microenvironment that is distinguished from that in
solution by confinement of solutes in the hydrogel pores. However,
hydorgels only mimic biological extracellular matrices to some
extent as the hydrogel fiber organization lacks any order, unlike
it is in the case of the cholesteric liquid phase, e. g. chitin.
The hydrogel strength is adjustable by changing its solid content.
It further increases local hydrogel fiber co-aligments that to some
extent will mimic organic matrices in biological hard tissues.
Different kinds of hydrogels were used to study calcite
crystallization (silica, agarose, gelatin). As each hydrogel has
different characteristics, hydrogels can act differently in
promoting or inhibiting crystallization. Hydrogels have an ability
to mechanically impede the growth of a crystal depending on the
strength of the hydrogel. Gelatin hydrogel is a poly-peptide
material derived from natural collagen through hydrolytic
degradation. The hyrolitic degradation breaks the triple-helix
structure of collagen into single-strand molecules. Gelatin
contains both acidic and basic amino acids with isoelectric point
values near ∼5 and with predominance of acidic moieties. Agarose
hydrogel is a linear polysaccharide extracted from marine red
algae. It consists of beta-1,3 linked D-galactose and alpha-1,4
linked 3,6-anhydro-alpha-L-galactose residues. Gelatin and agarose
hydrogels are composed of a fibrous structure that have varying
mesh void dimensions depending on the hydrogel solid content.
Hydrogel with 2.5 wt % gelatin solid content exerts less pressure
against the growing calcite crystal aggregate than a hydrogel with
10 wt % gelatin solid content. Silica hydrogel does not exert
strong pressure against the growing calcite crystal aggregate due
to its nature as it is composed of minute (less than 20 nm) sized
spherical particles that do not appear to form a network. The
hydrogel strength together with the growth rate of the crystal
defines the amount of incorporated hydrogel into the growing
calcite crystal aggregate such that a strong hydrogel will
incorporate more gel into the calcite crystal than a weak hydrogel.
Calcite grown in Mg-free silica hydrogels has a rhombohedral shape
and is elongated on the c-axis. It grows as dumbbell-shaped
aggregates in the presence of Mg. Silica hydrogel either Mg-free or
Mg-bearing does not give a major influence on the co-orientation of
the obtained crystal aggregate. Calcite grown in Mg-free agarose
has two morphologies: rhombohedron-shaped calcite crystals and
calcite radial aggregates. Calcite grown in Mg-bearing agarose has
sheaf-like and peanut like morphologies. The presence of Mg in
agarose influences the co-orientation of calcite crystals within
calcite Mg-bearing agarose composites. The calcite/Mg-free agarose
composite has several large crystal subunits while the
calcite/Mg-bearing agarose composite shows a spherulitic
microstructure. In the case of gelatin hydrogel, the precipitate
consists of calcite aggregates that have a variety of features i.e.
the formation of mosaic crystals and mesocrystal-like subunits in
one aggregate, the formation of aggregates with a fan-like
distribution of the c-axis orientation and the formation of
spherulitic aggregates. The formation of aggregates with different
characteristic in the subunits can be explained as a result of a
combination between local differences in gelatin matrix arrangement
and physicochemical conditions such as the change in Mg/Ca ratio,
pH, saturation, etc. The development of a fan-like distribution of
the c-axes orientation in the calcite aggregate subunits can be
explained as a result of Mg intrasectorial zoning. A different
degree of Mg incorporation in different growth steps will
accumulate misfit strain in the lattice. This misfit strain could
be released through the formation of dislocations at regular
intervals, such that small-angle boundaries develop. This growth
further leads to the extreme split growth and the formation of
fan-like and spherulitic crystal aggregates. The etching
experiments of calcite/hydrogel composites reveal the structure of
the incorporated hydrogel within the calcite crystals and
aggregates. In the case of Mg-bearing silica hydrogel more silica
hydrogel is incorporated into the calcite crystal than in the case
of Mg-free silica hydrogel. Thick hydrogel membranes are observed
when Mg-free gelatin and agarose hydrogels are used. These
membranes do not occur when Mg is present. The formation of these
membranes in Mg-free gelatin and agarose hydrogels is a result of
an accumulation of the hydrogel fibers that are driven back by
growing crystals or aggregates. The stiffness of the gelatin and
agarose hydrogel fibers increase as Mg is added into the hydrogel.
The hydrogel becomes stiffer and exerts more pressure against the
growing aggregates. No hydrogel membranes are observed in
aggregates grown in Mg-bearing gelatin and in agarose hydrogels. On
the basis of biopolymer and mineral composites, gypsum
(CaSO4)/cellulose fiber composites were prepared. The purpose of
the addition of cellulose fiber to gypsum was to create a composite
with a high ecological value and interesting mechanical properties
such as high Young’s modulus, high bending strength and high
compression strength. The cellulose fiber affects the mechanical
property of the composites depending on the fiber characteristics,
e.g. the nature of the cellulose (natural or synthetic), water
retention value, degree of swelling, etc. Lyocell fiber, a
synthetic fiber, is found to be able to increase the Young’s
modulus of the final composite.
Mytilus edulis, are composites of biopolymers and minerals. M.
edulis has two distinct layers, the outer layer consists of fibrous
calcite and the inner layer is composed of nacreous aragonite.
Close to the interface between nacreous aragonite and fibrous
calcite, a 1-2 micrometer wide zone exists that consists of
granular aragonite. Aragonite granules and tablets as well as
calcite fibrous are embedded into matrix biopolymers. In order to
understand the composite nature of these hard tissues, biomimetic
experiments using hydrogels were carried out. Hydrogels are able to
model biogenic matrix environments due to their ability to confine
space and to determine diffusion rates, local concentrations and
supersaturation of the solutes. Hydrogels have local
crystallization microenvironment that is distinguished from that in
solution by confinement of solutes in the hydrogel pores. However,
hydorgels only mimic biological extracellular matrices to some
extent as the hydrogel fiber organization lacks any order, unlike
it is in the case of the cholesteric liquid phase, e. g. chitin.
The hydrogel strength is adjustable by changing its solid content.
It further increases local hydrogel fiber co-aligments that to some
extent will mimic organic matrices in biological hard tissues.
Different kinds of hydrogels were used to study calcite
crystallization (silica, agarose, gelatin). As each hydrogel has
different characteristics, hydrogels can act differently in
promoting or inhibiting crystallization. Hydrogels have an ability
to mechanically impede the growth of a crystal depending on the
strength of the hydrogel. Gelatin hydrogel is a poly-peptide
material derived from natural collagen through hydrolytic
degradation. The hyrolitic degradation breaks the triple-helix
structure of collagen into single-strand molecules. Gelatin
contains both acidic and basic amino acids with isoelectric point
values near ∼5 and with predominance of acidic moieties. Agarose
hydrogel is a linear polysaccharide extracted from marine red
algae. It consists of beta-1,3 linked D-galactose and alpha-1,4
linked 3,6-anhydro-alpha-L-galactose residues. Gelatin and agarose
hydrogels are composed of a fibrous structure that have varying
mesh void dimensions depending on the hydrogel solid content.
Hydrogel with 2.5 wt % gelatin solid content exerts less pressure
against the growing calcite crystal aggregate than a hydrogel with
10 wt % gelatin solid content. Silica hydrogel does not exert
strong pressure against the growing calcite crystal aggregate due
to its nature as it is composed of minute (less than 20 nm) sized
spherical particles that do not appear to form a network. The
hydrogel strength together with the growth rate of the crystal
defines the amount of incorporated hydrogel into the growing
calcite crystal aggregate such that a strong hydrogel will
incorporate more gel into the calcite crystal than a weak hydrogel.
Calcite grown in Mg-free silica hydrogels has a rhombohedral shape
and is elongated on the c-axis. It grows as dumbbell-shaped
aggregates in the presence of Mg. Silica hydrogel either Mg-free or
Mg-bearing does not give a major influence on the co-orientation of
the obtained crystal aggregate. Calcite grown in Mg-free agarose
has two morphologies: rhombohedron-shaped calcite crystals and
calcite radial aggregates. Calcite grown in Mg-bearing agarose has
sheaf-like and peanut like morphologies. The presence of Mg in
agarose influences the co-orientation of calcite crystals within
calcite Mg-bearing agarose composites. The calcite/Mg-free agarose
composite has several large crystal subunits while the
calcite/Mg-bearing agarose composite shows a spherulitic
microstructure. In the case of gelatin hydrogel, the precipitate
consists of calcite aggregates that have a variety of features i.e.
the formation of mosaic crystals and mesocrystal-like subunits in
one aggregate, the formation of aggregates with a fan-like
distribution of the c-axis orientation and the formation of
spherulitic aggregates. The formation of aggregates with different
characteristic in the subunits can be explained as a result of a
combination between local differences in gelatin matrix arrangement
and physicochemical conditions such as the change in Mg/Ca ratio,
pH, saturation, etc. The development of a fan-like distribution of
the c-axes orientation in the calcite aggregate subunits can be
explained as a result of Mg intrasectorial zoning. A different
degree of Mg incorporation in different growth steps will
accumulate misfit strain in the lattice. This misfit strain could
be released through the formation of dislocations at regular
intervals, such that small-angle boundaries develop. This growth
further leads to the extreme split growth and the formation of
fan-like and spherulitic crystal aggregates. The etching
experiments of calcite/hydrogel composites reveal the structure of
the incorporated hydrogel within the calcite crystals and
aggregates. In the case of Mg-bearing silica hydrogel more silica
hydrogel is incorporated into the calcite crystal than in the case
of Mg-free silica hydrogel. Thick hydrogel membranes are observed
when Mg-free gelatin and agarose hydrogels are used. These
membranes do not occur when Mg is present. The formation of these
membranes in Mg-free gelatin and agarose hydrogels is a result of
an accumulation of the hydrogel fibers that are driven back by
growing crystals or aggregates. The stiffness of the gelatin and
agarose hydrogel fibers increase as Mg is added into the hydrogel.
The hydrogel becomes stiffer and exerts more pressure against the
growing aggregates. No hydrogel membranes are observed in
aggregates grown in Mg-bearing gelatin and in agarose hydrogels. On
the basis of biopolymer and mineral composites, gypsum
(CaSO4)/cellulose fiber composites were prepared. The purpose of
the addition of cellulose fiber to gypsum was to create a composite
with a high ecological value and interesting mechanical properties
such as high Young’s modulus, high bending strength and high
compression strength. The cellulose fiber affects the mechanical
property of the composites depending on the fiber characteristics,
e.g. the nature of the cellulose (natural or synthetic), water
retention value, degree of swelling, etc. Lyocell fiber, a
synthetic fiber, is found to be able to increase the Young’s
modulus of the final composite.
Weitere Episoden

vor 9 Jahren
In Podcasts werben
Kommentare (0)